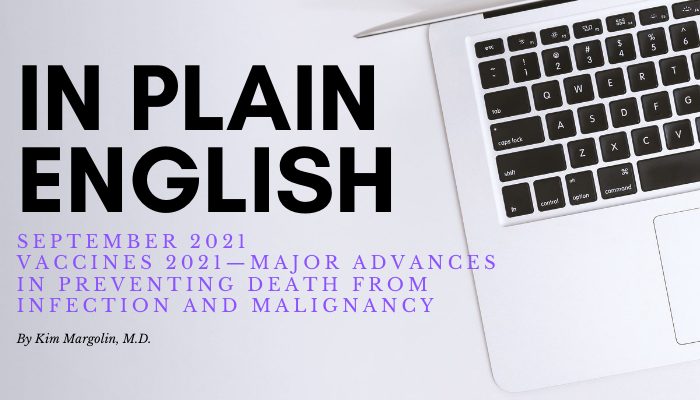
By Kim Margolin, M.D., FACP, FASCO
Vaccine background and brief history
The word “vaccine” comes from the Latin word vacca for “cow” and relates to early research by Edward Jenner that found people who had cowpox virus infection were protected against later smallpox infection. “To vaccinate” thus became the verb used to describe the process of using the material from one infectious organism to protect individuals from infection caused by the same or a related organism. Subsequent studies and usages have broadened the meaning, so that vaccinate/vaccination and immunize/immunization are now used interchangeably and generally understood to mean the process of administering a biological material into the body with the intent of preventing a specific illness. Most vaccines that we are familiar with are those that are intended to prevent future illness; these are called preventive vaccines. Newer research is focused on vaccines designed to cure or reduce the severity or complications of established infections or other illnesses, including malignancy; these are called therapeutic vaccines.
Vaccines can be divided into a number of different types—live-attenuated infectious organisms, mRNA (genetic material) wrapped in a shell of biological material to enhance its delivery and protect it from being digested, and particles from the organism the vaccine is intended to target, delivered inside of an unrelated virus. There are many other ways to make and deliver vaccine, but they all ultimately function the same way, which is to help the immune system recognize a pathogen (a disease-causing organism) or part of a pathogen (a protein called an antigen) as foreign so the immune system will mount a response to it. Our immune systems do a pretty good job of discerning many pathogens and antigens on their own, but vaccines help the immune response to act more quickly to recognize the specific pathogens and antigens that may be harmful if not quickly eradicated.
Preventive vaccines—the goal is to avoid infection
Preventive vaccines, such as those given to most individuals during childhood, are intended to induce an immune response that will block serious infections by various organisms (mainly bacteria and viruses) or decrease the seriousness of such infection if it occurs.
Examples of preventive vaccines include the nearly universal childhood administration of vaccines against measles, mumps and rubella, diphtheria, pertussis and tetanus, polio, influenza B, and several others. (You can find more information on these vaccines at the U.S. Centers for Disease Control subsite vaccinateyourfamily.org). Post-vaccination exposure to any of these organisms generally induces a highly protective and boosting immune response in your body, in most cases making it unnecessary to repeat the vaccine series or to receive a booster vaccination. But some of these vaccines do require boosting over time or in certain situations. For example, when a person is potentially exposed to tetanus as a result of a penetrating injury, a tetanus booster is recommended. Another example is influenza vaccination, which is recommended yearly due to the very high rate of mutation among strains of the influenza virus and the large number of annual deaths caused by these influenza strains.
Most preventive vaccines are not capable of causing illness, other than the typical vaccine reactions resulting from local pain at the injection site (nearly always in a muscle) and a general inflammatory reaction to one or more of the vaccine components—muscle aches, low-grade fever, fatigue—which tend to peak at one to two days after vaccination and then resolve.
Some preventive vaccines are live-attenuated, meaning that the organism itself is used to induce the vaccination, but that organism has been chemically or heat-treated to prevent it from causing fully-developed infection. Examples of live-attenuated virus vaccines include the childhood measles-mumps-rubella vaccine, rotavirus, smallpox, chickenpox, and yellow fever vaccines. These types of vaccine should not be given to people whose immune systems are weaker than normal, because in this case the attenuated organisms may actually cause infection.
The COVID-19 Vaccines—the newest preventive vaccines
Currently, there are two types of preventive COVID-19 vaccines used in the U.S., based on the method they use to deliver the virus genetic material that induces the immune response against Coronavirus: messenger RNA (mRNA) and adenoviral vector. Both of these vaccine types deliver the nucleic acid (mRNA or DNA) that gives our cells instructions for how to make a harmless antigen—a piece of the so-called “spike protein” from the surface of the COVID-19 virus. Once we are vaccinated, if we are exposed to the virus, our immune system can quickly recognize that spike protein (immune memory) and begin producing large quantities of antibody that prevents the virus from sticking to proteins in our lungs, something that is required to establish serious infection.
The mRNA vaccines deliver the genetic code to our cells in a nanoparticle of lipid (a VERY small piece of fatty material). Both the Pfizer and the Moderna vaccines are made from mRNA. Vector vaccines deliver the genetic code to our cells using a different (not COVID), harmless virus as a vector (which means vehicle). This adenovirus has been engineered so that it cannot make you sick; it merely serves as a vehicle for the instructions.
These vaccines made from nucleic acids (mRNA or DNA) are not live-attenuated vaccines—the Coronavirus is not injected into a person’s body, which means that you cannot get COVID from the vaccine. Similarly, the vaccine will not alter your own genes.
Bridging the gap between preventive vaccines and future cancer
There is a small group of preventive vaccines that can actually reduce the risk of selected cancers caused by certain viral infections that cause permanent alterations of our cells and tissues, leading to cancer development many years after the viral infection. Often, the viral infections themselves are silent, causing no symptoms—for example, the human papilloma virus (HPV) strains that cause cervical or head and neck cancer. Others include hepatitis B vaccines to prevent liver cancer, which kills about 400,000 people globally each year. These preventive anti-cancer vaccines, if widely distributed to people at risk (such as adolescents prior to sexual activity in the case of HPV), could potentially save hundreds of thousands of lives worldwide each year. Other vaccines with the potential to prevent cancers resulting from infection—such as Helicobacter pylori and Merkel Cell Carcinoma—may soon be possible, based on current research. Ongoing investigations will also help to inform vaccine developers about the critical genetic and other factors that cause certain infected individuals but not others to develop cancer, so that vaccination efforts, costs and resource utilization can be focused on at-risk individuals.
The next frontier—moving toward the use of therapeutic vaccines in immunotherapy strategies against established cancer
Attempts to vaccinate people already diagnosed with cancer have been ongoing for many decades but have been met with remarkably low rates of success. There are many important and seemingly insurmountable causes for this failure to eliminate established cancer by vaccination. One overarching barrier to achieving an effective and safe anticancer vaccine is the intrinsic property of the human immune system that evolved to distinguish foreign antigens (threats to human well-being or survival, such as viruses and other infections) from “self-antigens”—those that result from the body’s normal handling of its own natural proteins and other cellular substances that belong in our bodies. In most cases, this ability to detect the difference between foreign and self-antigens is what protects us. However, when tumors develop, they always carry foreign substances resulting from mutations or other cell damage responsible for alterations that could be recognized and attacked by our immune system. The problem is that because the vast majority of tumors develop very slowly over time, the immune system develops a “tolerance” to the abnormal substances and fails to attack the cells that should look foreign. That is why researchers often use the term “breaking tolerance” to describe the goals of tumor vaccines or tumor immunity. Among the other major challenges to effective antitumor vaccines is the problem that some antigenic materials on tumor cells are also found on normal cells—again, the immune system will normally be tolerant to these substances until a vaccine is developed that breaks tolerance. In this case, however, breaking tolerance also unleashes the immune system to attack normal tissues, which can be dangerous for the patient.
Of course, not all tumor vaccines are based on viruses, and many tumor antigens are well-understood and already making some progress on their way to effective antitumor vaccines. The methods for tumor antigen delivery in these vaccines may be very complex and involve, as in the case of a prostate cancer vaccine, the use of other immune cells (dendritic cells) that are obtained from the patient, treated with an immune-enhancing blood cell growth factor plus the vaccine material, and then given back to the patient. This vaccine was developed for men with prostate cancer and a rising PSA, which is a blood test that measures indirectly the growth of prostate cancer cells. The PSA vaccine, known as Sipuleucel-T (Sip-T) and commercially as Provenge, has shown activity in delaying the time to prostate cancer symptoms such as bone pain or need for other treatments and enhances the average lifespan of treated patients. The current version of Sip-T also became a basis for developing more active vaccines that are currently in trials, such as adding more potent immune cell growth factors to the vaccine preparation.
Many other approaches to therapeutic vaccine development have been studied, many of which use tumor-derived whole proteins rather than mRNA or DNA such as were detailed above for the current virus vaccines. When whole proteins are used, our immune systems are able to use them in many ways to stimulate immunity against the tumor antigens, and there are fewer restrictions on the patient’s immune system characteristics than when vaccines are produced from tiny antigenic fragments of the tumor-derived protein. The sources of protein are quite broad and include whole tumor cells taken from the patient him/herself or from other patients with the same tumor but treated with radiation or chemicals to make them unable to survive in the patient and to make them more immunogenic (able to provoke an immune response). It is also possible to get tumor antigens by washing them off the tumor cell surface with special solutions and then purifying them to use only the proteins with antigenic properties.
Although the field of tumor vaccines really started in large part with melanoma, because of its longstanding known immune responsiveness and its historically poor response to other treatments, it is remarkable that to date, no tumor vaccine has succeeded in meeting the level of activity needed for approval in melanoma. Furthermore, with the advent of highly-active immune checkpoint blockade (ICB), discussed in a previous edition of In Plain English—the need for vaccine therapies has changed, and the strategies are now directed at enhancing current results with ICB by adding vaccines to approved and investigational ICB antibodies. Furthermore, technical improvements in our understanding of melanoma antigens have paralleled the recent work that led to the rapid development of nucleic acid-based Covid-19 vaccines in late 2020, and initial studies in melanoma have shown great promise for these types of vaccine, which are based on the patient’s unique, mutation-based melanoma antigens delivered as mRNA in nanoparticles. Because these vaccines are truly unique to tumor mutations that were critical for the malignancy to develop and may be required for the tumor to maintain its malignant behavior, this type of vaccine is the most likely to be highly tumor-specific and to spare the normal tissues, thus enhancing its safety.
Additional ways to essentially vaccinate patients against tumor antigens have been discussed in a previous edition of In Plain English, when the subject of intratumoral therapy was reviewed. While the approved intratumoral agent talimogene laherparepvec (TVEC, brand name Imlygic) is not designated as a vaccine per se, TVEC and some of the other intratumoral agents currently under investigation actually do work like vaccines in facilitating the presentation of tumor-derived antigenic material to immune cells, resulting in the stimulation of an immune response against a tumor that is expected to work locally but also to sensitize immune cells that will go into the circulation and eliminate tumors at non-injected sites.
The last decade has truly been the decade of immunotherapy, with melanoma patients in the vanguard, benefiting most from a huge number of scientific and clinical advances. There is still a long way to go in melanoma and other malignancies, but thanks to the enthusiasm and hard work of investigators in the lab, the clinic and both, patients at risk and those with established melanoma are likely to benefit even more from continued immunotherapy advances in the foreseeable future.
Kim Margolin, M.D.
Dr. Margolin is a medical oncologist specializing in melanoma and other skin cancers. She worked at City of Hope for 30 years and also held faculty positions at the Seattle Cancer Care Alliance/University of Washington and at Stanford University. Among her academic achievements were long-term leadership of the Cytokine Working Group, leadership involvement in the Cancer Immunotherapy Trials Network, participation in the Southwest Oncology Group’s Melanoma Committee, and many positions in the American Society of Clinical Oncology and the Society for Immunotherapy of Cancer. Dr. Margolin has reviewed grants for many cancer-related nonprofit organizations and governmental agencies. She has also served as a member of the Oncology Drugs Advisory Committee to the FDA, the American Board of Internal Medicine’s Medical Oncology certification committee, and the Scientific Advisory Committee of the European Organization for the Research and Treatment of Cancer. Among her research interests are brain metastasis in melanoma and immunotherapy of skin cancers. Dr. Margolin currently collaborates with surgeons and laboratory investigators at Saint John’s Cancer Institute and leads the institute’s efforts in melanoma/skin cancer clinical trials.
Dr. Margolin collaborates with AIM at Melanoma to write the monthly “in Plain English” to provide timely updates on new developments for patients, caregivers, and other individuals with an interest in medical advances in melanoma.
Recent Posts

From a Scare to Awareness: Lindsay Willis’s Mission to Educate on Melanoma Risks

Everything Coming up Rosy is not a Health Tune

Ways to Give: How You Can Donate Your Birthday Through Facebook

Beyond the Clinic Podcast: Navigating the Storm: Managing Stress and Anxiety Through Your Cancer Journey
